What Is Quantum Chip Testing? Key Insights and Techniques
2025.04.18 · Blog
As quantum computers edge closer to practical utility, testing quantum chips has become a critical step in scaling up reliable, error-resilient hardware. From ensuring high gate fidelity to minimizing error rates, quantum chip testing involves complex protocols that directly impact performance and scalability.
In this article, we dive into why quantum chip testing is essential, what parameters are evaluated, and how rigorous validation is pushing quantum computing toward the next frontier.
What Is a Quantum Chip Test?
A quantum chip test refers to the process of evaluating the performance, stability, and quality of qubits fabricated on a quantum processor. These tests are essential to:
-
Verify the functionality of qubits
-
Measure coherence times
-
Assess gate fidelity
-
Identify error rates
-
Ensure chip reliability under controlled experimental conditions
This process is especially vital during R&D, production, and before integrating chips into quantum systems or cloud platforms.
Why Quantum Chip Testing Is Crucial
Unlike classical chips, quantum processors operate using fragile quantum states that are highly susceptible to decoherence and noise. As such, testing is a critical step in the quantum chip development cycle. Comprehensive testing of quantum chips yields multiple advantages:
-
Ensuring Qubit Quality: Identifying and mitigating defects that can lead to decoherence and computational errors.
-
Validating Performance Metrics: Measuring coherence times, gate fidelities, and readout accuracies to assess qubit functionality. -Optimizes qubit performance before deployment in real-world systems.
-
Facilitating Scalability: As quantum processors scale to include more qubits, testing ensures that increased complexity doesn't compromise performance.
-
Accelerating Development: Rapid testing cycles enable quicker iterations in design and fabrication, speeding up the path to practical quantum computers.
-
Provides benchmark data for cross-platform comparisons.
-
Guides error correction protocols by quantifying actual system noise.
Robust testing helps researchers and manufacturers pinpoint weaknesses and continuously refine architectures.
Key Parameters Evaluated in Quantum Chip Testing
Modern quantum chip test platforms evaluate multiple physical and logical metrics. The most critical among them include:
1. Qubit Coherence Times
Coherence time reflects how long a qubit maintains its quantum state. Two main types are tested:
-
T₁ (Relaxation Time): Time a qubit takes to decay from |1⟩ to |0⟩. Measures how long a qubit retains energy in its excited state before decaying to its ground state.
-
T₂ (Dephasing Time): Indicates how long a qubit maintains phase coherence while in a superposition state.
Longer coherence times indicate more stable qubits and better computation.
2. Gate Fidelity
Gate fidelity quantifies how accurately quantum logic gates (like X, H, CNOT) are implemented. Quantum chip testing helps identify any deviation from ideal behavior due to noise, calibration errors, or fabrication defects.
-
Low gate fidelity results in computational errors.
-
High-fidelity gates (typically above 99.9%) are necessary for fault-tolerant quantum computing.
Testing includes randomized benchmarking and quantum process tomography to precisely measure gate performance.
3. Readout Fidelity
This refers to how accurately the quantum system can measure the state of a qubit.
-
Inaccurate readouts degrade algorithmic reliability.
-
Readout errors can be systematic (bias toward 0 or 1) or random (thermal noise, crosstalk).
High readout fidelity is essential for reliable quantum computation and error correction.
4. Error Rates
Error rate is a comprehensive measure of system noise and gate imperfections. It typically includes:
-
Single-qubit gate error: The probability of incorrect state evolution during single-qubit operations.
-
Two-qubit gate error: Often higher than single-qubit errors due to increased complexity and qubit interactions (e.g., CNOT gates).
-
Measurement error: Errors introduced during the final state readout phase.
Error rates are a limiting factor for quantum volume, circuit depth, and overall reliability. They are key inputs for fault-tolerant threshold calculations and quantum error correction schemes.
5. Crosstalk Analysis
Crosstalk refers to unwanted interactions between qubits. Crosstalk occurs when the operation on one qubit unintentionally affects another, especially in densely packed qubit arrays. Quantum chip tests detect and mitigate crosstalk to ensure individual qubits operate independently when needed.
Testing for crosstalk involves:
-
Driving qubit A and observing induced phase shifts or flips in qubit B.
-
Calibrating control pulses and isolating pathways.
Minimizing crosstalk is essential for scaling multi-qubit systems.
6. Thermal and Environmental Stability
Quantum chips, which typically operate at cryogenic temperatures, are extremely sensitive to environmental factors such as temperature fluctuations, magnetic fields, and mechanical vibrations. Testing evaluates how these conditions impact the chip's performance over time.
-
Superconducting qubits typically operate at 10–20 millikelvin using dilution refrigerators.
-
Stability tests ensure qubit behavior remains consistent over time and across environmental shifts.
Why Is Quantum Chip Testing So Challenging?
Quantum chip testing is significantly more complex than classical chip testing due to the delicate nature of quantum systems and the unique characteristics of quantum information. The key challenges include:
1. Fragile Quantum States
Quantum bits (qubits) exist in superpositions and entangled states, which are highly sensitive to external disturbances. Even the smallest interaction with the environment—like thermal noise, electromagnetic fields, or stray vibrations—can collapse or decohere the quantum state, making testing extremely delicate.
2. Cryogenic Operating Conditions
Most quantum chips, especially those based on superconducting or spin qubits, must operate at ultra-low temperatures (millikelvin range). Testing equipment must function reliably under cryogenic conditions, requiring specialized infrastructure like dilution refrigerators and low-noise measurement lines.
3. Measurement Limitations
Quantum measurement is inherently probabilistic and destructive—observing a quantum state collapses it. To characterize a qubit accurately, many repeated measurements are required under identical conditions, leading to long data acquisition times and statistical challenges.
4. Crosstalk and System Complexity
As the number of qubits increases, so does the complexity of interactions between them. Crosstalk between control lines or qubits introduces unwanted correlations that are difficult to isolate during testing. Verifying multi-qubit gates and entangled states requires sophisticated calibration and error modeling.
5. No Direct Debugging
Unlike classical systems where outputs can be directly inspected and traced, quantum systems offer no straightforward way to "peek inside." Testing relies on indirect techniques like quantum tomography, randomized benchmarking, and process fidelity analysis, which are mathematically and computationally intensive.
6. Error Characterization and Noise Modeling
Quantum error sources (e.g., dephasing, relaxation, control errors) are subtle and interdependent. Accurately characterizing single-qubit gate errors, two-qubit gate errors, and readout errors requires complex statistical models and can be hardware-specific.
SpinQ Offers Quantum Chip Testing and QPU Characterization Services
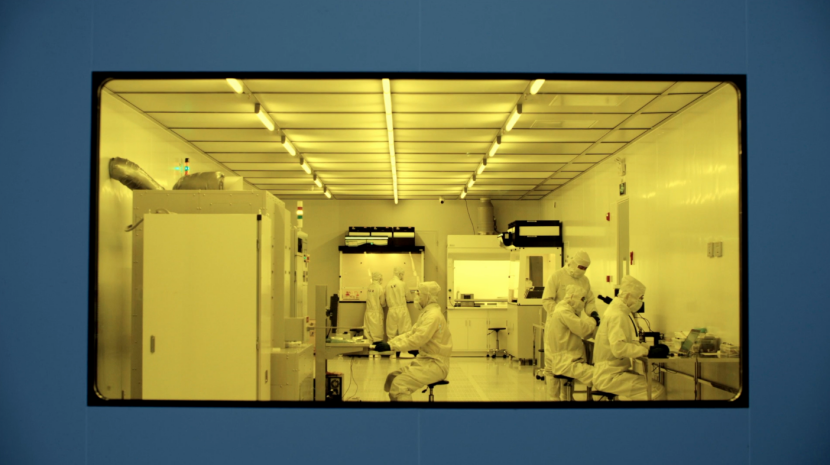
Are you looking for reliable testing solutions to support your quantum research or quantum computing applications? SpinQ is here to help.
We provide comprehensive quantum chip testing and QPU characterization services, tailored to diverse testing needs. Our evaluations cover key quantum performance metrics, including:
-
Coherence time measurement
-
Gate fidelity benchmarking
-
Error rate analysis (single-qubit gates, two-qubit gates, and measurement errors)
Standardized and Comprehensive Test Reports
SpinQ delivers detailed and standardized test reports with every quantum chip. These reports include essential parameters such as:
-
Qubit design specifications
-
Resonant cavity frequency
-
qubit frequencies
-
Decoherence times
-
Readout fidelity and crosstalk levels
-
Quantum gate performance
At SpinQ's QPU testing center, we also offer customized testing services, including quantum algorithm verification and performance optimization under specific application scenarios.
These services empower our clients to fully understand their chip's real-world quantum capabilities and ensure optimal performance across scientific and industrial applications.
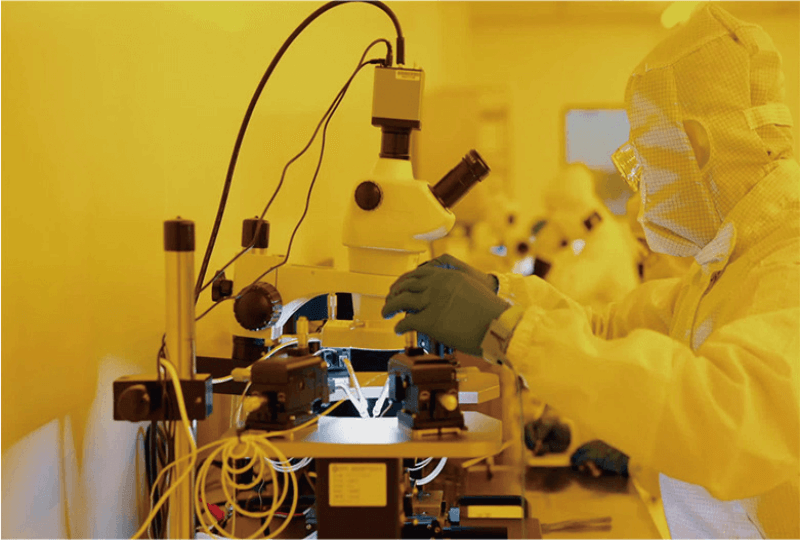
Quantum Chip Testing in Industry: Trends and Use Cases
Companies like IBM, Google, and Microsoft are heavily investing in quantum chip testing to advance their quantum computing initiatives. For instance, IBM's Quantum Characterization Lab rigorously tests chips like Eagle and Heron, ensuring they meet stringent performance standards before deployment.
Startups such as Orange Quantum Systems and QuantaMap are developing specialized testing equipment and methodologies to address the unique challenges of quantum chip evaluation, contributing to the maturation of the quantum hardware ecosystem.
FAQs
What are the quantum chip testing techniques and tools?
Modern quantum chip testing employs sophisticated methods and equipment:
-
Cryogenic Testing: Utilizing dilution refrigerators to cool chips to millikelvin temperatures, minimizing thermal noise and preserving qubit coherence.
-
SQUID-on-Tip Scanning: Employing superconducting quantum interference devices (SQUIDs) mounted on atomic force microscopes to detect minute magnetic fields and temperature gradients, revealing defects and inconsistencies in chip fabrication.
-
Automated Test Equipment (ATE): Implementing systems like OrangeQS MAX, capable of testing over 100 qubits simultaneously, significantly reducing testing time and increasing throughput.
-
Quantum Diagnostics Libraries: Integrating software tools that facilitate rapid analysis and interpretation of test data, aiding in quick decision-making during development.
How does IBM test quantum processors?
IBM conducts quantum processor testing in its Quantum Characterization Lab, where chips like Eagle and Heron undergo rigorous evaluation before deployment. The testing process involves placing the chip in a dilution refrigerator to achieve cryogenic temperatures necessary for quantum operations.
Researchers then measure key performance metrics such as qubit frequency, coherence times (T₁ and T₂), and gate fidelities. These assessments ensure that each processor meets stringent quality standards for reliable quantum computation.
For a detailed overview, you can watch IBM's lab tour video on YouTube.
What company is making quantum computer chips?
This article highlights the top 5 companies in 2025 leading the quantum chip industry, showcasing their pioneering technologies and contributions to quantum computer chips and the quantum technology revolution.
How does a quantum chip work?
A quantum chip uses quantum bits—or qubits—to perform computations based on the principles of superposition and entanglement. Unlike classical bits, qubits can represent 0 and 1 simultaneously, allowing quantum chips to solve certain problems much faster than traditional processors. These chips operate at ultra-low temperatures and require precise control of quantum states using microwave pulses or laser systems.
Check out this ultimate guide for a complete breakdown of how a quantum chip works.
Is Google's quantum chip real?
Yes. Google unveiled Willow, a 105-qubit superconducting quantum processor, in December 2024. Willow achieved a major breakthrough by performing a benchmark computation in under five minutes that would take a supercomputer 10 septillion years, demonstrating exponential error reduction as the system scales.
Is Microsoft's quantum chip real?
Yes. In February 2025, Microsoft introduced Majorana 1, the world's first quantum processor powered by topological qubits. This chip utilizes a novel material called a topoconductor to create more stable and scalable qubits, aiming to build a million-qubit quantum computer capable of solving complex industrial problems in the future.
Has anyone built a quantum computer yet?
Yes. Explore this comprehensive guide featuring 18 leading quantum computer manufacturers, showcasing their cutting-edge technologies, hardware innovations, and how they are shaping the future of quantum computing.
Does Elon Musk have a quantum computer?
No, Elon Musk does not currently possess a quantum computer. While he has expressed significant interest in the field, particularly in the integration of quantum computing with artificial intelligence, he has not developed or announced a proprietary quantum processor.
Featured Content