What Is Superposition in Quantum Computing? Expert Explained
2025.04.23 · Blog
Quantum Superposition in Quantum Computing: From Physics to Processing Power
Superposition is one of the most fascinating and fundamental principles in quantum computing. It's what gives quantum computers their unique power—and sets them apart from classical machines.
But what exactly is superposition? How does it work, and why is it central to the revolution that quantum computing promises?
This article offers a deep dive into the concept of superposition, covering its physical intuition, mathematical representation, and role in real quantum algorithms.
What Is Quantum Superposition? A Quantum State of Coexistence
In classical computing, a bit can be either 0 or 1. That's it. Everything your laptop or phone does is based on combinations of these two states.
Quantum computing uses qubits (quantum bits). Unlike classical bits, qubits can exist in a superposition of both 0 and 1 at the same time. This is not just flipping quickly between the two states—it's a blend of both until you measure it.
Think of a spinning coin. While it's in the air, it's not strictly heads or tails—it's both. That's a rough analogy for superposition.
Mathematically, a qubit in superposition is written as:
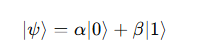
Here, ∣0⟩ and ∣1⟩ are basis states, and alphaα and betaβ are complex numbers representing probability amplitudes. When you measure the qubit, it will "collapse" into either 0 or 1, but before that, it holds the potential for both.
Visualizing Superposition: The Bloch Sphere
To better understand superposition, physicists often use the Bloch Sphere, a geometric representation of a qubit state. On the Bloch Sphere:
-
The north and south poles represent the classical states ∣0⟩ and ∣1⟩
-
Any other point on the sphere corresponds to a unique superposition of ∣0⟩ and ∣1⟩
This allows infinite combinations, not just two possibilities, greatly expanding the information a single qubit can encode before measurement.
Quantum Superposition and the Double-Slit Experiment
The most famous demonstration of superposition is the double-slit experiment.
When individual particles such as electrons are fired at a barrier with two slits, they form an interference pattern on the detection screen—only explainable if each particle travels through both slits at once, in superposition.
If a measurement device is placed to observe which slit the particle goes through, the interference pattern disappears. This shows that superposition is destroyed by measurement, collapsing the system into a definite state.
This experiment reveals the counterintuitive nature of quantum systems: particles behave like waves when unobserved and like particles when observed.
Superposition and Schrödinger's Cat
The thought experiment Schrödinger's Cat offers a vivid, if bizarre, illustration of superposition applied at the macroscopic scale.
Imagine a cat placed in a box with a quantum mechanism: if a radioactive atom decays, the cat dies; if it doesn't, the cat lives. According to quantum theory, until we open the box, the cat is in a superposition of alive and dead states.
While not a literal physical experiment, it emphasizes a core quantum idea: quantum systems exist in multiple states until measured—an idea that carries profound implications for quantum computing.
Creating Superposition: The Hadamard Gate
One of the most common ways to create a superposition is by using a Hadamard gate (H-gate). When applied to a qubit in state ∣0⟩, it transforms it into:
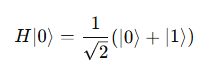
This creates a perfectly balanced superposition with equal probability amplitudes—often used as the starting point in quantum algorithms like Grover's search and Shor's algorithm.
What Is Superposition in Quantum Computing?
In quantum computing, superposition allows quantum bits (qubits) to represent both 0 and 1 simultaneously.
A classical bit is always 0 or 1. But a qubit in superposition:
can hold a continuum of values between 0 and 1 (within quantum constraints), making it exponentially more powerful in certain types of computation.
For an n-qubit system, superposition enables the representation of 2ⁿ different states at once. For example, 3 qubits can represent all 8 states from ∣000⟩ to ∣111⟩ simultaneously—not sequentially, but in a parallel quantum state space.
Superposition vs. Classical Parallelism
It's tempting to think of superposition as just "parallel processing", but it's far more nuanced.
-
Classical bits can simulate parallelism by using multiple processors.
-
Qubits in superposition can encode 2ⁿ states simultaneously for an n-qubit register.
However, you can't extract all this information at once. Quantum computers use interference to amplify correct answers and cancel out incorrect ones, which is the basis for quantum algorithmic advantage.
Physical Realization of Superposition
Superposition isn't just theoretical—it's been experimentally verified in:
-
Superconducting qubits (used by IBM, SpinQ, and Google)
-
Trapped ions (used by IonQ and Quantinuum)
-
Photonic qubits
-
Neutral atom systems
In all these systems, delicate engineering is required to maintain coherent superposition states against decoherence, the tendency for quantum information to be lost due to environmental interaction.
Why Superposition Matters in Quantum Computing
Superposition is not just a fascinating physics concept—it's the foundation of quantum computational power. Unlike classical bits that can only be in one state (0 or 1), qubits can exist in a superposition of states, allowing quantum computers to explore a vast solution space simultaneously.
Thanks to superposition, quantum computers can:
-
Process multiple outcomes at once through quantum parallelism
-
Perform complex calculations in parallel, accelerating problem-solving
-
Handle exponentially larger data sets than classical systems
Imagine trying to crack a password from a million possibilities. A classical computer tests each option one by one. A quantum computer, leveraging superposition, could evaluate many of those possibilities simultaneously—dramatically speeding up the process.
Moreover, the power of superposition is amplified when combined with other quantum phenomena:
-
Interference helps amplify correct answers while cancelling out incorrect ones
-
Entanglement works synergistically with superposition to process correlated information across qubits
These quantum properties enable breakthrough algorithms such as Shor's algorithm (for factoring large numbers) and Grover's algorithm (for searching unsorted databases), solving problems that are practically impossible for classical computers.
Real-World Applications of Quantum Superposition
Thanks to quantum superposition, quantum computing is already moving beyond theory and into real-world applications.
Quantum computers are now being used—or seriously piloted—in sectors like:
1. Cryptography: Superposition allows for the execution of algorithms like Shor's algorithm, which can factor large numbers exponentially faster than classical algorithms—posing both a challenge and opportunity for modern cryptographic systems.
2. Drug discovery: By simulating molecules in superposition, quantum computers can evaluate multiple molecular configurations at once, making it possible to discover new drug candidates more quickly and accurately. Companies like Roche and Pfizer are actively exploring this field.
3. Financial modeling: Superposition enables simultaneous analysis of thousands of market scenarios, improving portfolio optimization and risk analysis. Financial institutions like JPMorgan Chase and Goldman Sachs are investing in quantum computing R&D.
You May Be Interested: How Quantum Computing Benefits Financial Services
4. Artificial intelligence: In quantum machine learning, superposition allows models to process and learn from high-dimensional data spaces far more efficiently than classical methods. Techniques like quantum-enhanced feature mapping are being tested in image recognition and NLP.
5. Climate modeling: Quantum computers can simulate climate systems with a huge number of interacting particles, helping scientists better understand weather patterns, ocean behavior, and long-term climate shifts.
To explore more practical use cases of quantum computing in various industries, check out our in-depth article on real-world use cases of quantum computing.
Challenges and Misconceptions of Quantum Superposition
-
Superposition doesn't mean a qubit holds both 0 and 1 values like a memory card. It's a quantum probability landscape that only resolves upon measurement.
-
Measurement destroys superposition, collapsing the qubit into a definite state.
-
Noise and decoherence limit how long a superposition can be maintained, a major hurdle in building large-scale quantum systems.
Final Thoughts
Quantum superposition isn't just a theoretical curiosity—it's the reason quantum computing exists. From the mystery of electrons passing through two slits to the cutting-edge algorithms of today's quantum processors, superposition connects the abstract world of quantum mechanics with real-world technological breakthroughs.
As we build larger and more stable quantum systems, mastering superposition will be key to unlocking the future of computation.
Featured Content